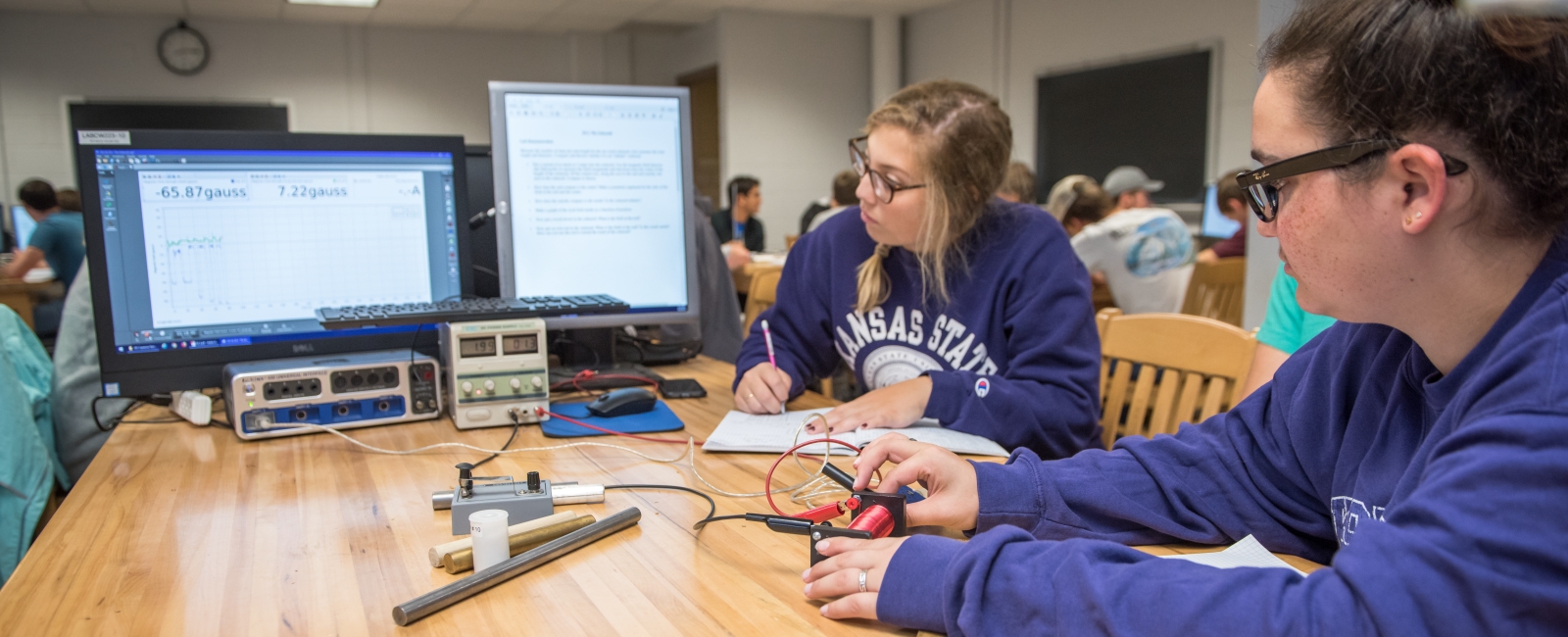
Department of Physics
Advancing physics through excellence in teaching and research so that people can understand the world around us, inside of us, and beyond us
The Department of Physics at Kansas State University is built around highly productive faculty members with teaching and research expertise. Research is conducted in our department and other world-class facilities such as Fermi National Lab, CERN, DESY (German Electron Synchrotron), SLAC National Accelerator Laboratory, Lawrence Berkeley National Lab providing students a wide variety of experiences.
A full length (05:10) video is available on our K-State Physics YouTube channel.
Recent News and Announcements
- Spring 2024 alumni newsletter available
- Kansas State University promotes 70 faculty members including Daniel Rolles, 25 receive tenure
- Physicists publish research on ultrafast dynamics in a molecular photoswitch in Nature Chemistry
Customizable curricula for your career interests and ambitions
Advisors and mentors to support you academically and professionally
Access to first-class research facilities here and around the world
Meet Our Accomplished Faculty
Our faculty conduct research in atomic, molecular and optical physics, in condensed, soft and biological matter physics, in cosmology and high-energy physics, and physics education.