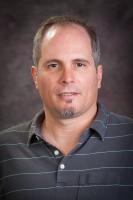
Radio Frequency Electrochemical Crystallization—Crystal Growth One Atom at a Time
The process of crystal growth can be divided into three general classes—ideal growth, surface kinetics-limited growth, and diffusion-limited growth, with diffusion-limited growth being the least understood and the least applied in scientific and industrial ventures (e.g. protein structural analysis, pharmaceutical purification). In fact, the broader crystal growth community by and large disregards diffusion-limited crystallization because the resulting crystals tend to be heavily branched, defect-ridden, and weaken the integrity of bulk crystals. Over the past ~10 years, my group had studied radio frequency (RF) electrochemical crystallization, attaining a basic picture of this diffusion-limited, electrochemical process and developing a number of applications ranging from neural probes to plasmonic materials. RF electrochemical crystallization occurs on time scales near the minimum of what diffusive transport requires. Essentially, an RF voltage is applied to an electrode immersed in aqueous salt solution, cycling the electrode potential between +1.0 V and -1.0 V, ~40 million times per second. During a positive half cycle, metallic ions, such as AuIIICl4- for the case of gold crystallization, diffuse to the interface and adsorb onto the electrode surface. The average inter-ion distance d in a 40.0 mM salt solution is ~ 3.5 nm, so the electrode must wait approximately d2/2D ~ 5 ns for an ion to diffuse to its surface, where D is the diffusivity of the ion. A 40.0 MHz voltage signal has a half cycle duration of 12.5 ns. Hence, only a few atoms will diffuse to and adsorb onto the electrode surface during such a short half cycle period. During the subsequent negative half cycle, a fraction of the adsorbed ions are reduced to the crystalline metal (Au0), and the crystal grows (slightly). By varying the amplitude of the RF voltage signal, we control the magnitude of that fraction. If the amplitude is adjusted so that the growth velocity of the crystal is minimal but positive, many of the historical problems of diffusion-limited crystal growth are overcome. In this region of parameter space, we have grown branchless, ultra-long, single crystalline gold nanoribbons. We can also grow millimeter long, 400 nm diameter, branchless conducting polymer nanofibers, as well as a number of other kinds of structures. Applications for these nanowires will be discussed.