Electron Event Selection in Doubly Charged Higgs Simulations
Reese Sanders, Rochester Institute of Technology, Physics and English Major
Mentored by Dr. Andrew Ivanov
The Standard Model (SM) of Particle Physics is a vital tool for modern High Energy Physics. Despite the many valid predictions made by the SM, it is inherently flawed. When the SM was developed, it was under the assumption that neutrinos were massless, however experiments had deemed that to be false. The question becomes how we amend the Standard Model such that we explain the tiny neutrino masses found in experiments.
One set of explanations, Seesaw Models, posits that there is some particle with a high mass that couples with the neutrinos to give them such a small mass. We are most concerned with the Type II Seesaw Model which suggests that there exists a Higgs triplet, introducing a double charged Higgs Boson (DCH) into the SM. In one simplistic model, the mass parameter of the Higgs Triplet is the massive term that results in the light neutrino masses[1].
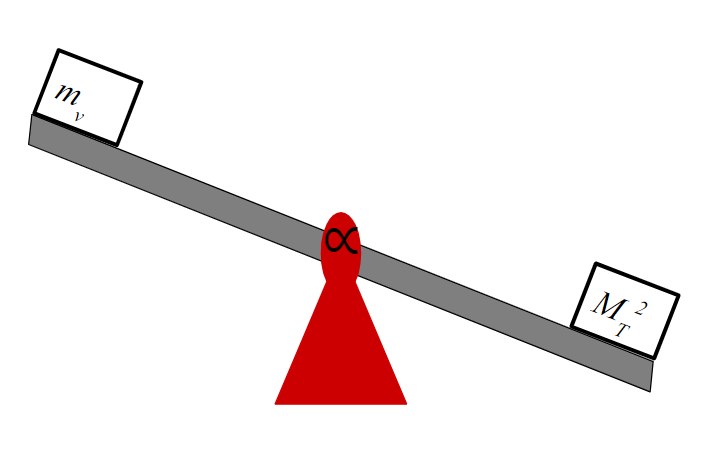
In this project, we used data from a Monte Carlo (MC) simulation to examine what parameters should be satisfied when looking for the DCH as well as how we can differentiate between the momenta of background interactions and the momenta of DCH decays. In these simulations we assume that the DCH decays into any two same sign leptons [2]. However, since we anticipate two DCHs of opposite signs, for reconstruction we need to identify four lepton candidates for reconstruction.
Since we chose to focus on electrons for this project, isolated events when the DCHs decayed into four electrons. This minimized the number of events we encountered with zero electrons detected. Following this event selection, we analyzed the efficiency of triggers and estimated the expected contribution of both background and DCH signals.
Events are registered for electrons if the triggers are satisfied. These triggers can be in the form of either the detection of a single electron or two electrons. A third trigger can be formulated by using both the single electron and double electron triggers. In Figure 2, we see that the most efficient use of our triggers is to apply both the single and double electron triggers.
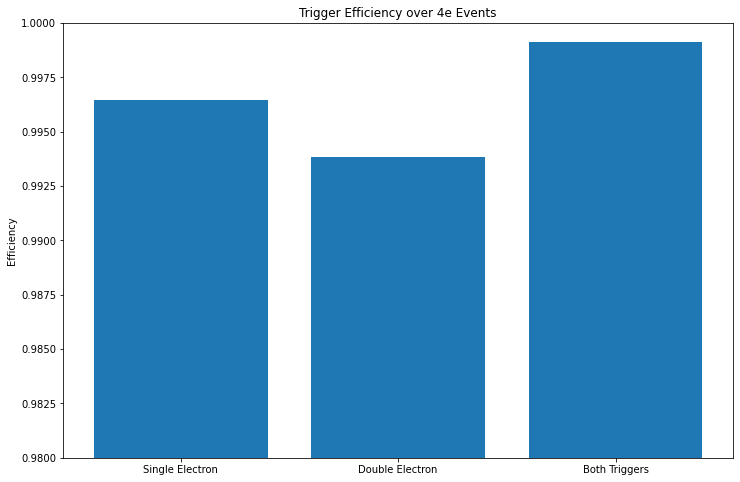
The cuts applied to both the signal and background data varied in their restrictiveness. We utilized five different cuts in our initial analysis (veto, loose, medium, tight, HEEP). In our analysis of the background, we focused on events where three electrons passed through the loose cut when a Z boson decays to an electron positron pair. In Figure 3, we can see the outcome of these cuts. All background events where there are at least three loose electrons have three loose electrons, while the signal is spread across several electron counts.
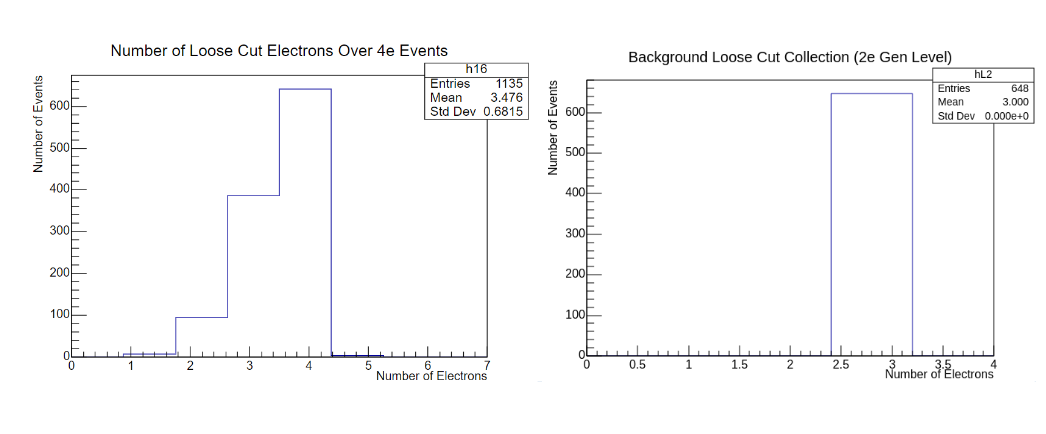
Using the counts determined in figure three, we can estimate the contribution of the background, and DCH signals in real data through a set of relatively simple equations.
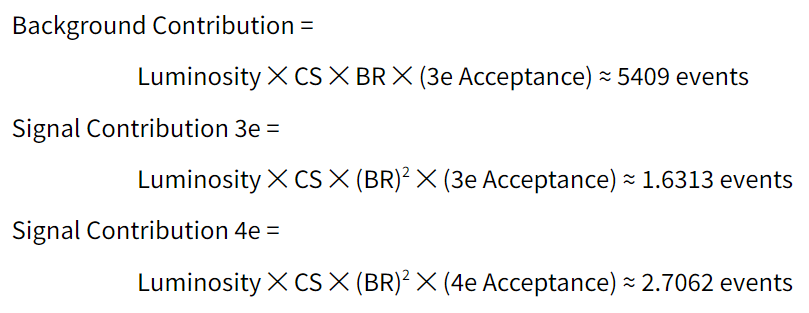
Where CS is the cross section of the decay, BR is the branching ratio, and the Acceptance is the number of loose 3e events (or 4e) divided by the total number of events. From this we expect that there will be very few three and four electron events, so we need to find a way to filter out this background almost entirely. The simplest way to do this is to adjust the momentum where we make our cuts. In Figure 5, the overlap between the background and signal is minimized around 200 GeV, therefore we should require electrons used in reconstruction to have greater than 200 GeV in their transverse momentum.
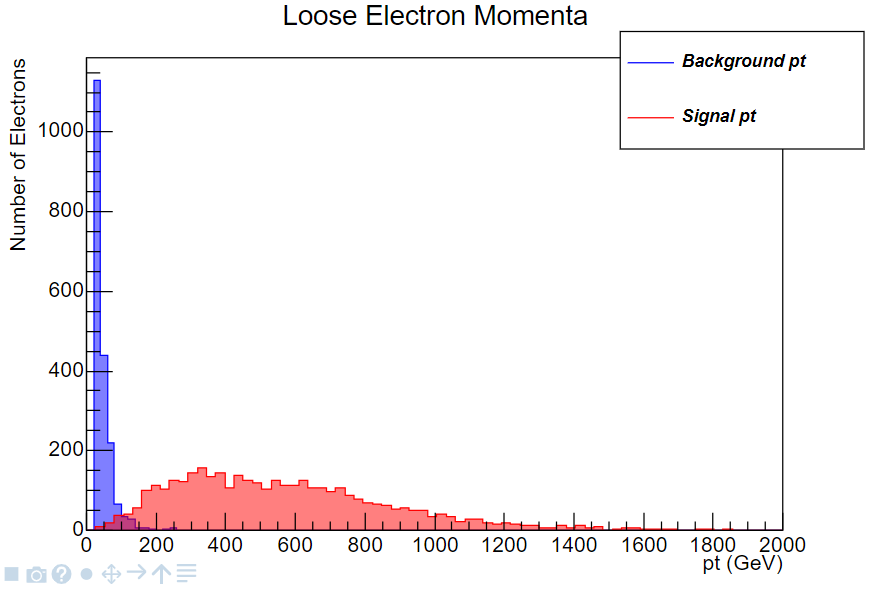
This had only been one background analyzed though. In the future we would want to repeat this analysis with other background and add up the expected contribution from each. Furthermore, this process should be accomplished with taus and address the mixed lepton decays of the DCH. With all of the backgrounds and leptons analyzed, the analysis process can be continued by searching for the DCH signal in the selected events.
References
[1] Stefan Antusch, et al., arXiv:1811.03476 [hep-ph] (2018), “Low scale type II seesaw: Present constraints and prospects for displaced vertex searches.”
[2] The CMS Collaboration, CMS Physics Analysis Summary., “A search for doubly-charged Higgs boson production in three and four lepton final states at √ s = 13 TeV”
Acknowledgements
I want to thank the National Science Foundation for funding the research as well as Kim Coy, Dr. Loren Greenman, and J.T. Laverty for putting together the entire program. More importantly, I would like thank Dr. Ivanov for taking me on as a mentee and giving me my first proper taste of high energy physics research.